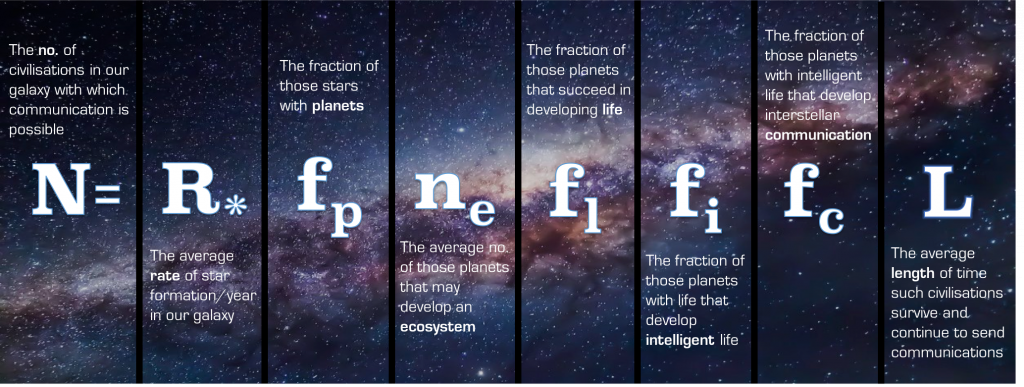
It is now 63 years since the late Dr Frank Drake published his now famnous and epononimous equation that bears his name, back in 1960 this was a very brave decision, whilst UFO fever was rife in the media and public, in the science community it was seen as a pseudoscience because we had no evidence of planets orbiting other stars, let alone life existing on them, back then, like now, we still do not know how life starts and under what conditions, but, in the 63 years since it’s initial publication, we have improved our understanding of the Universe, planets, life and can make better assessments of the functions within the Drake equation, and we can alter the Drake equation to give us a clearer idea, well we hope, on the preponderous of any form of life – although, unless it is in the Solar system and we can physcially find it, establishing that a given world plays host to life will be a never ending challenge.
So where do we start?
In this article we will refer to any object on which life may evolve as a world, because it is highly probable that life could start when that body is a moon of a giant planet, thus to refer to each object upon which we may find life as a planet could be misleading, and given that there is a possibility of life on, at least, moons in our solar system (Enceledus, Titan and Europa), we cannot ignore that possibility.
We need to remember that in 1960, humanity knew of no planets outside the solar system, but now we have confirmed the existance of 5574 Exoplanets (Exoplanets Database 2023 -12-29) with another 10,808 to be confirmed by follow up observations. As of the latest catalogue data, 3696 planets orbit stars within the mass range 0.6Mʘ and 1.499Mʘ (71%) and 703 (16.9%) of the 4138 stars with confirmed planets are confirmed as type V (dwarf main sequence hydrogen burning) stars – many of the stars do not have their spectral type and class confirmed, but many are likely to be type V (dwarf main sequence stars).
Thus, we now have vastly more information than we did back in 1960, there are 5574 confirmed planets with more than 10,000 more awaiting confirmation – it is likely that many more are in the existing data sets to be processed, more will certainly be found in the coming years in existing data and by the new resources that are coming online.
We need to determine which stars we should be looking at – stars are found across a huge mass range, with the smallest around 100th Mʘ and some well over 100Mʘ, the Sun actually sits toward the top end of the mass range and is certainly an above average star. The mass of the star is important – stars more mass than the Sun have considerably shorter lives, both on the main sequence and overall, the mass of the star is inversely proportional to its life span, so the higher the mass, the shorter the life – see table 1 below.
A star like the Sun can expect to live for around 10.5 billion years, with maybe 8 – 9 billion years on the main sequence, burning hydrogen, it is currently estimated to be around 5.3 billion years old, thus, about 50% of the way through it’s life, if we look at table 1 (below), you can see how the mass of the star impacts its life expectancy.
Table 1: The Stellar Population of the Milky Way and How Mass impact life Expectancy
Main Life Span Periods and Population Comparisons of the Stellar Population for the Milky Way Galaxy | ||||||
Mass | Main Sequence Life | Total Life | Average Effective Temperature | Luminosity | Estimated Percentage of Galaxy Population | Total Number of stellar population |
Sun = 1.0 | Billions of Years | 0K | Sun=1.0 | Based on Gaia & Hipparcus Data | Based on an estimate of 350 Billion Stars in the Milky Way | |
0.10 | 2686 | 3,160.00 | 2200 | 0.00010 | 80% | 280000000000 |
0.20 | 475.15 | 559.00 | 2400 | |||
0.30 | 171.7 | 202.00 | 2600 | |||
0.40 | 83.725 | 98.50 | 3000 | 0.00100 | ||
0.50 | 48.025 | 56.50 | 4400 | |||
0.60 | 30.43 | 35.80 | 4800 | |||
0.70 | 20.7315 | 24.39 | 5000 | 0.01000 | 16% | 56000000000 |
0.80 | 14.841 | 17.46 | 5200 | |||
0.90 | 11.05 | 13.00 | 5400 | 0.10000 | ||
1.00 | 8.67 | 10.20 | 5600 | 1.00 | ||
1.10 | 6.698 | 7.88 | 5800 | 1.0 - 2.0 | ||
1.20 | 5.3805 | 6.33 | 6000 | 2.0 - 3.0 | ||
1.30 | 4.4115 | 5.19 | 6100 | 3.0 - 4.5 | ||
1.40 | 3.672 | 4.32 | 6200 | 4.5 - 6.0 | ||
1.50 | 3.077 | 3.62 | 7500 | 6.0 - 8.0 | ||
2.00 | 1.4875 | 1.75 | 8500 | 8.0 - 10.5 | 3% | 10500000000 |
2.50 | 0.8585 | 1.01 | 10500 | 10.5 - 13.0 | ||
Millions of Years | ||||||
3.00 | 544.85 | 641.00 | 10,500 to 12,500 | 13 - 20 | ||
3.50 | 370.8975 | 436.35 | 20 - 100 | |||
4.00 | 265.625 | 312.50 | ||||
4.50 | 197.8715 | 232.79 | ||||
5.00 | 152.15 | 179.00 | ||||
5.50 | 119.8075 | 140.95 | ||||
6.00 | 96.05 | 113.00 | 1000 - 10,000 | |||
6.50 | 78.9055 | 92.83 | ||||
7.00 | 65.756 | 77.36 | ||||
7.50 | 55.165 | 64.90 | ||||
8.00 | 47.175 | 55.50 | 2% | 700000000 | ||
8.50 | 40.29 | 47.40 | 12,500 to 20,000 | |||
9.00 | 34.9775 | 41.15 | ||||
9.50 | 30.549 | 35.94 | ||||
10.00 | 26.86 | 31.60 | ||||
10.50 | 23.8 | 28.00 | ||||
11.00 | 21.182 | 24.92 | 10,000 - 100,000 | 1% | 350000000 | |
11.50 | 18.955 | 22.30 | ||||
12.00 | 17 | 20.00 | ||||
12.50 | 15.385 | 18.10 | ||||
13.00 | 13.94 | 16.40 | ||||
13.50 | 12.7075 | 14.95 | ||||
14.00 | 11.5855 | 13.63 | ||||
14.50 | 10.625 | 12.50 | 0.90% | 315000000 | ||
15.00 | 9.741 | 11.46 | ||||
16.00 | 8.2875 | 9.75 | 20,000 to 60,000 | |||
17.00 | 7.1315 | 8.39 | ||||
18.00 | 6.1795 | 7.27 | ||||
19.00 | 5.3975 | 6.35 | 150,000 - 1,000,000 | 0.10% | 35000000 | |
20.00 | 4.7515 | 5.59 | ||||
25.00 | 2.72 | 3.20 | ||||
30.00 | 1.7 | 2.00 | ||||
50.00 | 0.476 | 0.56 | 1,000,000 - 10,000,000 | |||
100.00 | 0.085 | 0.10 |
Lets look at a revision of the classic Drake Equation and see how it differs from the original.
N = R*, InRB*, fp, Ne, fl, fc.FCiv,FcEx, Ftsc, FcL, FCL
As with the classic Drake equation,
N = the number of civilisations we can expect to exist at this current era of time.
From table 1, you would, initially, be forgiven for thinking that the lower the mass the better the star as it will live considerably longer than other stars, thus life would have far more time to evolve, but this has some caveats, stars below 0.6Mʘ are often referred to as flare stars, this is because they can erupt without warning and release coronal mass ejections with energy levels several thousand times that of the Sun – and any planet hit by such a CME would have its atmosphere likely stripped and the surface irradiated – not conducive to a stable environment for life to develop and evolve. In fact, the Trappist 1 star (referred to as the Trappist 1) is known to have 7 planets, all are similar in size to Earth, but so far, observations have confirmed the innermost 4 planets have no atmosphere, with 2 of them orbiting in the “habitable zone” of the Trappist 1 star, which is a red dwarf of spectral type M8V, but with a mass only 0.08Mʘ (12.5% the Sun’s mass and physically only a little larger than Jupiter!).
The other problem with diminutive stars is that any planet that may have temperatures conducive to the development of life is likely to be tidally locked by the gravitational interaction between the planet and the star, meaning that only one face of the planet will face the star, possibly being broiled, whilst the “nightside” of the planet being in perpetual darkness and frigid temperatures depending on the chemistry and physics of the atmosphere which could spread heat from the day side to the dark side to balance temperatures – however, observation seems to be implying these planets will not have any atmosphere due to the flares the stars produce as already discussed above.
Given these constraints placed by the length of the star’s life at the top end, and the unpredicable and exteme activity of those at the lower end of the star scale, we should concentrate our search for life bearing planets upon which higher and intelligent life could evolve to stars from 0.6Mʘ to 1.49Mʘ, this limits us to around 16% of the stellar population of the Milky Way galaxy. This is a vast reduction from the estimated 350 billion stars thought to reside in the galaxy, but as the table shows, this is still a potential population of 5.6E+10, or 56 billion stars – not an insignificant number.
Now, whilst 56 billion stars is a lot of stars, not all may have planets, and not all may host life bearing worlds, now we have to look at the statistical probability for every part of the Drake equation.
- R* – the rate at which stars form within the galaxy. Estimates put this figure at about 3 solar masses form stars each year within the Milky Way – so we can assume, in an average year, several tens, to perhaps hundreds, of stars less than the mass of the Sun form with random numbers of larger stars – so, based on what we know today about star formation, and the observations above, lets put the number of stars forming in the galaxy annually, in the range 0.6Mʘ and 1.49Mʘ at 1.5.
- InRB* – this new funtion takes into account the number of stars that evolve off the main sequence onto the instability track toward the Red Giant Branch each year. This figure is surprising hard to pin down, the SIMBAD database has data on more that 17 million objects, but the Gaia archive has billions of objects which can be cross referenced with numerous other sources, but narrowing down certain data is a chore, the following table, Table 2, gives an idea of the make up of the stellar population of the galaxy by spectral type, gleaned from the databases that are available, and then narrowed for the percentage of each spectralk type is a Type V (Main sequence star). This is a guide only and the figures can be sharpened with the use of accurate database serach tools and possibly even AI – which we do not have available.
Spectral Type | Percentage of Galactic Population | Approximate population number number | Approximate number in Type V | ||
O | 0.50% | 1750000000 | 175000000 | ||
B | 1.50% | 5250000000 | 2625000000 | ||
A | 2.00% | 7000000000 | 8400000000 | ||
F | 3.00% | 10500000000 | 2730000000 | ||
G | 6.00% | 21000000000 | 7980000000 | ||
K | 21.00% | 73500000000 | 54390000000 | ||
M | 66.00% | 231000000000 | 189420000000 | ||
The data in table 2 needs to be narrowed further and ensure that the star numbers only account for stars in the mass range of 0.6Mʘ to 1.49Mʘ. It gets even more difficult when attempting to understand the age of a given star, the majority of stars do not have this level of detail available, but looking at the stars that do we would suggest that perhaps 2 stars each year evolve off the main sequence and into the instability strip leading to the red giant phase of stellar evolution, 0.5 of these will be within our desired mass range in all probability.
- fp – The fraction of stars with planets – as we are only concerning ourselves with the mid range mass stars, and based on observations over the last 30 years, we can reasonably state that, as a minimum, at least 70% of stars in this range have planets – this gives us a minimum figure of 39.2 billion stars.
- Ne – the number of those planets that have an environment that can develop a life bearing ecosystem. Here we are getting back into guesswork territory, as we have no information that gives any clues as to the prevalence of ecosystems, but, being conservative, lets suggest that only 1 out of every 100,000 worlds forms a life capable ecosystem – we end up with 3, 920,000 worlds currently with life capable ecosystems.
- fl – the fraction of life capable ecosystems which lead to life – again, this is a guess, but based on what we see on Earth, where life can find a way it does gives us a good idea that where there is a stable ecosystem that can support life, then life will develop, so lets put this figue at 50% – so we end up with a figure of 1,960,000 worlds that harbour some form of life.
- fc – adding in an additional function for complex life here, because many ecosystems that develop life may be constrained to life that is complex, may be intelligent, but environmental factors, such as being on an Ocean world, may prevent evolution to an advanced civilisation capable life. At this stage we should likely consider the age of the star depending on the complexity of the life we are seeking, on Earth, simple life emreged around 600 million years after the planet formed, but as far as we know, complex life did not appear until the Cambrian Explosion, some 550 million years ago, which is 3.6 billion years after the planet formed and around 3 billion years after life appeared, so we should constrain our searched to stars that are at least 1.5 billion years old, however, as stars age, they move off the main sequence and move onto the instability strip, which is likely to make inhabited planets uninhabitable, given the life expectancy of stars, and the need for metals to be presant, we should nto be looking at stars older than 8 billion years, thus we have a window of 6.5 billion years, from 1.5 billion to 8.0 billion years in age, mass dependent. This is still a huge number of stars and given that life appears to naturally evolve into ever more complex organisms we can assume that 80% of planets on which life starts evolves into complex life, then we have a figure of 1,568,000 worlds with complex life.
- FCiv – another addition to the standard Drake equation, FC is the function for life that forms complex and highly organised societies that have a basic interest in science, technical development and social controls that put them above other species on the planet – akin to humanity around 10,000 years ago. I would posit that where complex life evolves on worlds with the correct environment, it is a natural process to eventually create a species that will become dominant on that world, but it is likely only 25% of worlds with complex life that have the conditions where this happens, this gives us a figure of 392,000 worlds with dominant species that have or are capable of forming complex societies and cultures. It may be wise to account for the age of a star at this time, we know that life on Earth started about 600 million years after the planet formed, thus, depending on the life we are looking for, the star needs to be at least 1.5 billion years old for basic life, but for more complex life, it would need to be around 3.5-4 billion years old, then, we ensounter the issue of the life of stars, any star in the mass range of 0.6Mʘ to 1.49Mʘ older than 8 billion years old would be evolving away from the main sequences and evolving into a red giant.
- FcEx – this function deals with extintion level events that occur on a world before any species is able to evolve to a complex societal level with an understanding of science and technology – on Earth there have been around 20 major events with 6 global extinctions that eradicated bewteen 60% and 85% of life know to exist at that time. It is likely this is common and part of a natural process on worlds with life, and whilst it may hinder given species, it may also be an engine for rapid evolution as new species fill niches left empty by the extinction event, the question here is how many species, on the road to developing a complex and science, technology based society are wiped out before they can achieve it – this is a hard figure to conclude, but based on the history of life on Earth, I would suggest this figure could be as high as 50%, thus we have reduced our possible worlds to 196,000.
- Ftsc – changing the equation again, Ftsc represents those societies that evolve into ultra complex societies with a deep undertsanding of sciences and development of technology, including communications, that may make them detectable. There are many factors here we have to account for, and attempt to remove human bias, but what we can assume is that any species that becomes dominant on its home world will be roughly similar to humans in being a territorial, predatory omnivore, thus, internal conflict amongst groups is highly probable, we see this in all life forms on Earth that have social structures and are territorial – contrary to many “old wives tales”, intergroup violence is not unique to humans or even higher primates, thus it is unlikely to not exist in alien animal groups. The difficult question here is how many complex societies with then evolve to this stage, we only have the example of humanity, and this has had a very stacato evolution, we have been held back in development by our own actions, such as conflict and religion, although at the same time, conflict has also driven progress, but we have seen more delays caused by conflict than advances until the modern era, plus there have been natural disasters that has eraditcated whole societies and decimated civilisations, but that fact is, we got there, we perceverred and prevailed, as I am sure any advanced life will as long as it is not wiped out by nature, thus I would suggest we accept that this figure is a high percentage, 75% will, at some stage, reach a level of technical development similar to humanity today as a minimum – that means 147,000 worlds hold civilisations either approaching, at or more advanced than humanity currently is.
- FcL – this function is indicating the expected lifespan of a civilisation that is able to maintain science and technological development – this is hard, human civilations have varied from around 250 years to almost 3000 years, there is no definitive period over which a civilisation will last, Sir John Grubb, in his 1976 tretis “The Fate of Empires”, stated that empires appear to follow predicable paths of rise, a period of extended stability and then, often, a slow decline over a period of some 250 years, although some have fallen violently or catastrophically due to events outside their control. We cannot assume all such civilisation will undergo similar processes, although it is likely, thus we can guess that 50% of advanced civilisations fail and thus need to start again, perhaps putting them back many thousands of years or even stopping their further development dead in it’s tracks. With this in mind, we reduce our number by 50% to 73,500.
- FCL – this is similar to the above, but relates to natural disasters that may see the end of the civilation, such as an asteroid impact, natural events on the planet, changes to their star, such as evolving off the main sequences into the Red Giant Branch, biological changes that render the species extinct or even global diseases that wipe them out – this is an unsettling idea, because to acknowledge this threat for other species, means we must accept it for humanity, and what happens will depend on whether the species has become a multiplanet species in their own planetary system before such an even occurs – and here there are numerous factors that come into play that can prevent a species becoming multiplanet.
- The world is more then 1.5 times the mass of Earth so chemical rockets cannot generate sufficient thrust to loft significant payloads from the surface to orbit.
- The world is actually a satellite of a giant planet/brown dwarf in the system and there are no planets or other worlds close enough to allow habitation – perhaps the envirnment around the planet/BD is sufficiently toxic to make travel all but impossible.
- A sufficiently dence atmosphere that makes flight difficult, as it requires significantly far more energy to power a craft, but also it may make the night sky uninteresting, depending on the chemistry of the upper atmosphere.
- Part of a system that is in a cluster of stars that prevents a night time sky as we experience it and thus they have no knowledge of the wider Universe.
- The world may lack sufficient mineral resources to allow the construction of craft capable of space flight.
- Religious beliefs may make space flight impossible.
- Fear of alien life may prevent them from becoming a multiplanet species.
- Constant conflict may make space flight impossible as it does require coperation across many cultures to get the skillsets required.
We can ssume that natural disasters, direct action and other influences may constrain the number of species that become multiplanet, perhaps more than we realise, there are many factors that may yet stop humanity becoming a multiplanet species, despite the best efforts of Elon Musk and Dr Robert Zubrin, thus, we will conservativly state that only a small percentage achieve this and use 35% as our figure, so that would suggest that only 25,375 species have, to date, become a multiplanet species.
That seems like a very large number of species that may be a multiplanet species or at least have very advanced technologies, but then we need to take into account the scale of the galaxy.
The Milky Way Galaxy has an estimated volume of 7.85E+15 cubic light years, which is a staggering volume of space, but when we divide this by 25,375 that means each species will call home a volume of around 309,359,605,911.3301 cubic light years, this is a volume with a diameter of 81,385 light years – a rather large distance!
So what can we take from this?
Intelligent and technologically advanced aliens clearly exist based on the above calculations, but the fact is they are rather a long way away on average, but the problem is, the galaxy only has a visible diameter of between 100,000 and 120,000 light years, it is not a sphere, most of the stars in the galaxy exist in the disc, thus the concentration of civilisations would have to be significantly higher, the galactic disk is estimated to have a volume of some 650 billion cubic light years, thus, the figure comes down to around 25.6 million cubic light years per species, that is a volume of space enclosed by a sphere some 301 light years across – which is a far more reasonable and workable figure, but it still encompasses around 300,000 stars that we know of.
Based on data the from the Exoplanet Catalogue, which currently has 5645 confirmed exoplanets listed, within 301 light years there are 3067 confirmed planets – most are certainly unlikely to support life, or at least life as we can imagine it, and many are significantly above the thresholds we have used in this review, but it is likely that for every planet we know of, there are likely 4-7 more that we have yet to ascertain, many of these may be within the right size range (0.7 to 1.5 Earth masses), orbiting a star between 0.6Mʘ to 1.49Mʘ and at a distance upon which liquid water may exist on the surface.
So where does this leave us, well let us assume that of the 3067 planets confirmed within 301 lights years, they represent 10% of the real number, so around 30,670 planets actually exist within 301 lights years, thus, if we then assume only 2% will match the criteria we need, the correct mass, the correct stellar parent, the correct environmental conditions etc, we are left with 613 planets within a radius of 301 light years that could, theoretically, support life as we know it – however, life as we know it does not automatically mean a technologically advanced species – life will likely evolve intelligence as a matter of evolution, we see this in many species once we take off our human blinkers, OK, so they cannot build or fly an aircraft, but nore can most humans -so it does not make them less intelligent, however, if we just look for those species that may have the abilitity to develop or have developed technology, we get into very murky waters as we only have an example of 1 – humanity.
So, how can we determine if any of these possible 613 planets have technical civilisations on them, that is the hard question…or is it?
Humanity thinks of intelligence as something humans possess and other animals do not, but in recent decades this view is being upturned, with species in many animal groups now being considered to have intelligence, contrary to the old wives tail, many birds are far from bird brained. The Corvids (Members of the Crow family), the Parots, birds of prey and several other groups have been shown to be able to learn, count and even use tools, many have excellent memory abilities too, equally, many fish species are a lot smarter than we give them credit, trout can learn, sharks have large brains and several species show clear intelligence, many fish species learn and can be taught, with memory capabilities equalt to that of cats and dogs, possibly even the higher order primates.
Lets take a look at the animal kingdoms of Earth.
Animal Group | Estimated Species | Estimated % of Intelligent species | Rough number of Intelligent species |
---|---|---|---|
Birds | 11,000 | 5 | 550 |
Mammals | 6,400 | 8 | 512 |
Insects | 10,000,000 | 2 | 200,000 |
Reptiles | 10,000 | 3 | 300 |
Fish | 40,000 | 5 | 2000 |
Molluscs | 50,000 - 200,000 | 1 | 500-2,000 |
How we define intelligence is crucial here, clearly there are no species of insect that are challenging humanity in the intelligence stakes, but this does not mean they are not intelligent, the same is true for all non-human species. However, where this is useful is gaining some level of estimation for how likely a technical species is to evolve on a given planet upon which complex life has arisen.
Given the seeming preponderance of life to evolve toward intelligence, especially the more complex that life becomes, from the table estimates above we can say that Earth is home to around 205,000 species that are “intelligent” to one level or another, if we then reduce this down further to those that have been demonstrated to solve complex problems, we can reduce this number to about 20,000 species overall, but if we then contrain this further, perhaps the number will drop to about 500 species – the number depends on how stringently you constrain the intelligence of an animal. However, if we get really conservative, we could constrain “real intelligence” to mammals, that means that we will have approximately 50 species that are truly intelligent, some likely more intelligent than humans, but not technologically capable. What this means is that technically advanced species have around a 1/50 chance of evolving on a given planet with complex life given sufficient time and a stable environment.
So, if we have about 613 planets within 301 light years upon which intelligent life may have evolved, that means that within that same radius, we have a 1/50 chance of finding a technical civilisation – possibly as many as 12 worlds – the question then becomes how many are less advanced than humanity, how many at a similar level, how many more advanced, and how many still exist!!
Now this really is the realm of pure speculation, bordering on science fiction to be sure, we have no way of knowing the answers to these questions, life on Earth does not afford us any more speculation down this avenue, obviously if the civilisation is pretechnological, they have died out or regressed backwards – we cannot detect them, if they are more advanced, we may just be able to detect evidence of them, but this is another wide open question and the proof would need to be concrete to be accepted, realistically, we would need to detect a civilisation at a similar technological level to humanity to be sure it was not natural, the chances of a civilisation, within 301 light years of Earth, being at a similar technological level, is vanishingly small – even then, communication will be all but impossible.
The conclusion we can draw from this is that the chances of finding a technologically advanced civilisation (at least equivilent to where we are now) within the 301 light year radius of Earth is vanishingly small, humanity was likely not alone in this bubble of space which encompasses around 300,000 stars, but stars orbit the galaxy and so they move apart, civilisations come and go in relatively small time frames, perhaps as little as a few hundred Earth years, some will not yet be advanced enough to detect or to detect us, and some may be significantly more advanced, depressingly the conclusion is that we may never know of any technically advanced species with any definitive conclusions.